FDRC Seminar Series
Each semester, FDRC invites speakers from across the United States and abroad to present their research on fluid dynamics to members of the Penn State community. Topics include fundamental research on turbulence, numerical methods for CFD, the development of experimental techniques, and engineering applications related to medicine, propulsion, combustion, and more.
Seminars are accompanied by complimentary coffee and donuts!
Fall 2023 Series
Seminars in this series are hosted every Thursday at 9:30 am in 125 Reber Building.
Schedule
Date | Speaker | Affiliation | Host |
---|---|---|---|
Aug. 31 | Chris Roh | Cornell University | Margaret Byron |
Sep. 7 | Leland Tien | Pennsylvania State University | Robert Kunz |
Sep. 14 | Christopher Combs | University of Texas at San Antonio | James Coder |
Sep. 21 | Lawrence Ukeiley | University of Florida | Adam Nickels |
Oct. 5 | Roberto Fernández | Pennsylvania State University | Samuel Grauer |
Oct. 12 | Jun Liao | Westinghouse Electric Company | Gregory Banyay |
Oct. 19 | Rui Ni | Johns Hopkins University | Samuel Grauer |
Oct. 26 | Dennice Gayme | Johns Hopkins University | Mark Miller |
Nov. 2 | Tracy Mandel | University of New Hampshire | Margaret Byron |
Nov. 9 | Ryan Murray | North Carolina State University | Zachary Berger |
Nov. 16 | APS Prep Session | Pennsylvania State University | |
Nov. 23 | Thanksgiving | ||
Nov. 30 | Colleen Witzenburg | University of Wisconsin–Madison | Melissa Brindise |
Dec. 7 | Michelle Driscoll | Northwestern University | Nathan Keim |
Abstracts and Biosketches
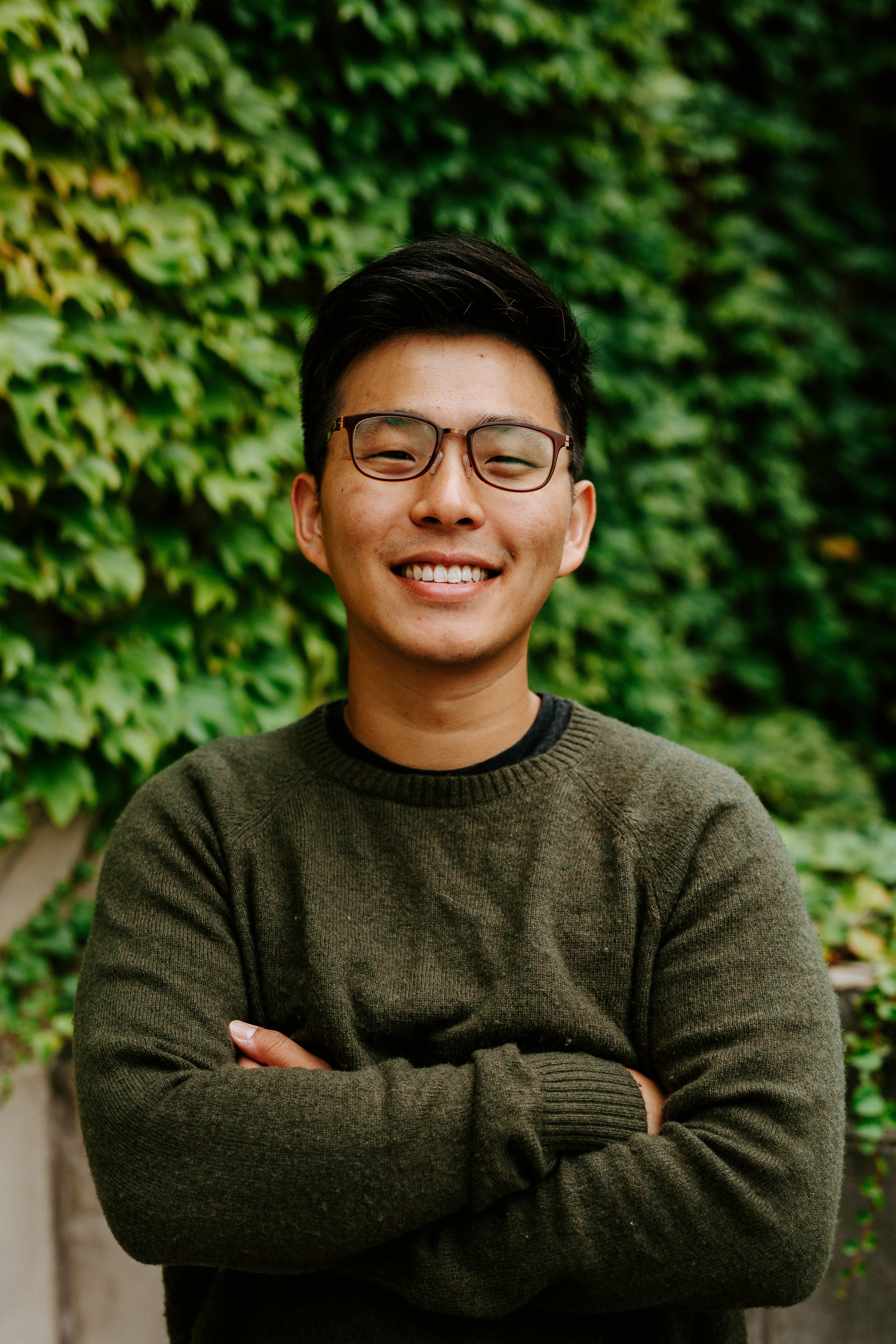
Chris Roh
Assistant Professor, Department of Biological & Environmental Engineering
Cornell University
Biological lift force
August 31, 2023
The profound impact of lift force on human travel is mirrored by its vital role in animal locomotion. In this talk, I will share two uses of lift force in biology in the context of flying and fast swimming.
I will first present flapping flight from the point of view of vortex formation. A recurring feature in animal locomotion is a roll-up of free shear layers into swirling vortical structures. During these roll-up processes, animals experience unsteady aerodynamic forces associated with the forming vortex, which can augment the force production. By considering vorticity flux and maximum circulation in the growing vortex, the dimensionless vortex formation time (inverse of Strouhal number) is generalized. The newly defined dimensionless vortex formation time associated with flight kinematics of wide set of biological flyers fell within a narrow range, pointing to a potentially a strong evolutionary selection pressure to maximize the leading edge vortex circulation.
The second part of the talk will be about the lift-based thrust of a whirligig beetle. The whirligig beetle is the fastest swimming insect, with forward swimming speeds reaching 100 body lengths per second. Previous studies have suggested that these beetles use a purely drag-based thrust to achieve their impressive swimming speed; however, our newly observed leg kinematics of the free-swimming beetle indicate drag-based thrust is used only in the early part of the power stroke and that lift-based thrust kicks in for the second half of the stroke. This observation is in-line with observed patterns in mammalian swimming, in which faster-swimming organisms rely on lift-based thrust generation to generate speeds not attainable through drag-based means alone.
Biosketch
Dr. Chris Roh received his B.S. in Biological Engineering from Cornell University in 2012 and his M.S. and Ph.D. in Aeronautics from the California Institute of Technology in 2013 and 2017, respectively. Chris has been fascinated by the diversity of insects and the different stories each tells. This deep-rooted passion, combined with a more newly found love for intricate fluid flows, led him to study the hydrodynamics of insects at Caltech under the guidance of Professor Morteza Gharib. He is now an Assistant Professor at Cornell University in the Biological and Environmental Engineering department. As the principal investigator of the in vivo Fluid Dynamics Lab, Chris continues to observe 'life in moving fluids' with engineering applications in mind. He is a recipient of the National Science Foundation Graduate Research Fellowship and the Richard B. Chapman Memorial Award.
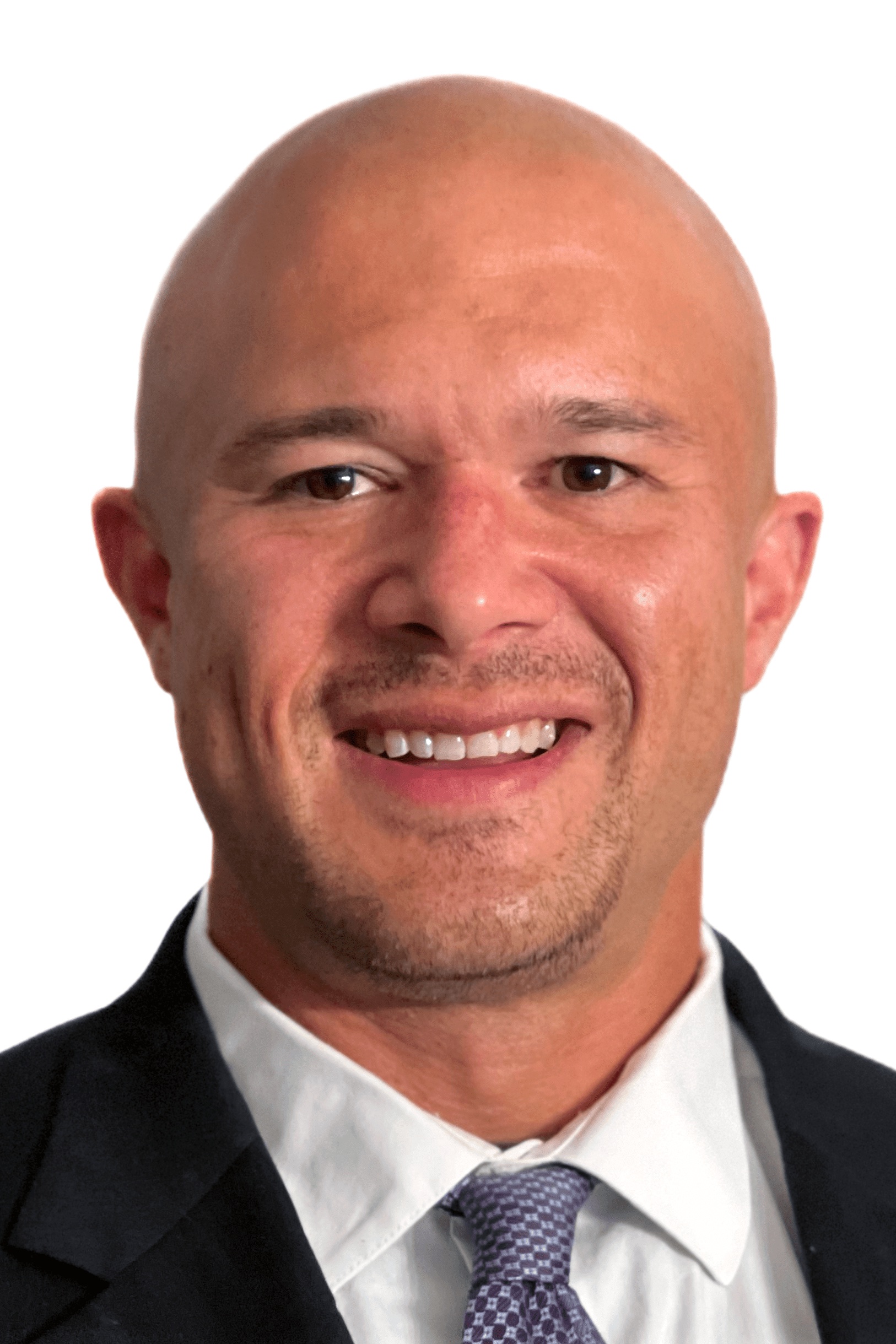
Leland Tien
Ph.D. Candidate, Department of Mechanical Engineering
Pennsylvania State University
CFD predictions and validation of the National Experimental Turbine Stage
September 7, 2023
Gas turbines have become the platform technology for modern society's energy and travel needs. The rising cost of fossil fuels coupled with the environmental need to reduce our carbon footprint make advancements in turbine design more important than ever. The National Experimental Turbine (NExT) program was created to address this problem. NExT is a government–industry–university collaborative effort to create an experimental test bed for improving gas turbine design.
Although experimental data is the gold standard in fluids research, computational fluid dynamics (CFD) provides many advantages and enrichment over experiments, alone. Once validated, CFD can offer i) a much higher resolution of the physics, ii) return of immeasurable parameters, and iii) the ability to explore operating conditions outside the scope of experimental limitation. This work seeks to leverage state of the art methods in CFD in the NExT experimental program to glean a better understanding of CFD methods and flow physics in gas turbines. The computational studies presented in this talk cover two primary topics. First, an assessment of a hierarchy of methods applied to pre-test predictions of NExT is covered. Second, current work on aerodynamic validation including incorporation of as-built airfoil geometry derived from structured light scans is presented.
Biosketch
Mr. Leland Tien is a Ph.D. candidate in the Department of Mechanical Engineering at the Pennsylvania State University, advised by Dr. Robert Kunz in the Computational Multiphase Physics laboratory. Mr. Tien is also employed part time as a research engineer at Penn State's Steady Thermal Aero Research Turbine laboratory. His dissertation is focused on applied turbomachinery CFD. He received his undergraduate degree in Engineering Science from Penn State.
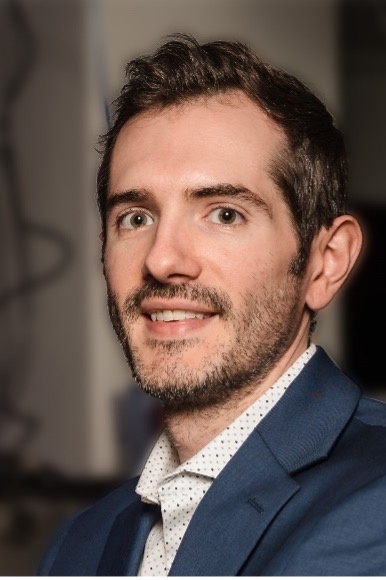
Christopher Combs
Dee Howard Endowed Associate Professor in Aerodynamics, Department of Mechanical Engineering
University of Texas at San Antonio
Applications of optical diagnostics in the UTSA hypersonic Ludwieg tube
September 14, 2023
This presentation will provide an introduction to the emerging hypersonic experimental research capability at UTSA (particularly focused on hypersonic aerodynamics and non-intrusive diagnostic development). Completed in 2021, the UTSA Mach 7 Ludwieg tube is a unique facility within American universities—one of only six at a U.S. academic institution operating at a Mach number of 7 or higher and one of only two with the current combination of high-Reynolds number (up to 200×106 m–1) and high-Mach number capability. The facility is also unique in that UTSA students were heavily involved in its design and construction. This has empowered the students to take responsibility for day-to-day facility operations, thus providing an excellent hands-on learning experience. The added availability of high-speed diagnostics equipment at UTSA makes the experimental test capability exceptionally rare.
Biosketch
Dr. Christopher Combs is the Dee Howard Endowed Associate Professor in Aerodynamics in the UTSA Department of Mechanical Engineering, where he is the Director of the Aerospace Engineering program, Director of the Center for Advanced Measurements in Extreme Environments, Director of the UTSA Hypersonics Lab, and Associate Dean Fellow for Research in the Klesse College of Engineering & Integrated Design. In the UTSA Hypersonics Lab, Combs leads a research group of over 20 graduate and undergraduate students studying problems related to hypersonic aerothermodynamics. He holds a B.S. degree in Mechanical Engineering from the University of Evansville and a Ph.D. in Aerospace Engineering from The University of Texas at Austin, where he was a NASA Space Technology Research Fellow. His primary area of research interest is in the development and application of non-intrusive laser-based measurement techniques for compressible flows and he has extensive experience in investigations of hypersonic flow physics, with over 50 technical publications in this field and over $16M in research funding from various organizations including NASA, USAF, NSF, US Navy, JHTO, and DARPA. Recognized as a recipient of the prestigious National Science Foundation CAREER and Air Force Office of Scientific Research Young Investigator Program awards, Dr. Combs led the design and construction of the new Mach 7 wind tunnel facility at UTSA. He is a senior member of the American Institute of Aeronautics and Astronautics and is also active with the American Society of Mechanical Engineers and the American Physical Society. Dr. Combs serves as a board member for the Dee Howard Foundation and is also a member of a variety of technical and service committees including the AIAA Aerodynamic Measurement Technology Technical Committee, the San Antonio Chamber of Commerce Aerospace Committee, and the Dee Howard Foundation Education Advisory Council.
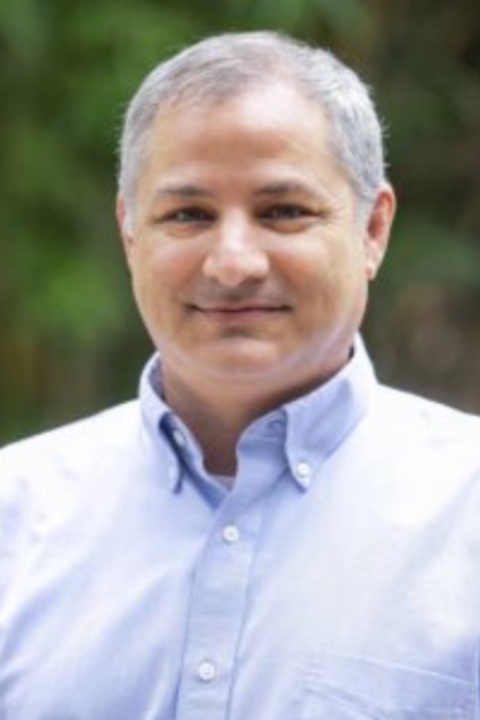
Lawrence Ukeiley
Professor, Department of Mechanical and Aerospace Engineering
University of Florida
High speed flow over open cavities and its control
September 21, 2023
The flow over open cavities represents a rich flow field and one of significant practical importance in the aerospace industry as well as many others. Fundamentally, the flow features in the cavity are driven by the shear layer at the opening and its interaction with the aft wall. These interactions create an intense aeroacoustic environment in and around the cavity, which can be detrimental in many of the applications. This presentation will highlight predominantly experimental studies of the characteristics of flow over two- and three-dimensional cavity geometries and attempts at control with the aim of reducing the surface pressure fluctuations. The studies of the flow characteristics have been conducted over a range of free stream Mach numbers and have involved both Particle Image Velocimetry and time-resolved surface pressure fluctuations measurements. These measurements have been used to develop low-dimensional descriptions of the flow using modal analysis and flow estimation techniques resulting in a time dependent description of the flow field. From these results and the introduction of some collaboratively performed numerical simulations, a better understanding of the sources in the flow has been developed which can be used guide flow control. In addition to the studies of the flow features open loop control using several leading edge blowing concepts.
Biosketch
Dr. Lawrence Ukeiley works in the fields of fluid mechanics, experimental methods, flow control, reduced order modeling, and fluid–structure interactions. He is currently a Professor in the Department of Mechanical and Aerospace Engineering at the University of Florida and serves as the Associate Chair for Academic Programs. He received his B.S. in Mechanical Engineering from Alfred University in 1989 and his M.S. and Ph.D. in Mechanical Engineering from Clarkson University in 1992 and 1996, respectively. Since graduating with his doctorate, he has held positions at BEAM Technologies (Research Scientist), NASA Langley Research Center (NRC Post-Doctoral Associate) and the Jamie Whitten National Center for Physical Acoustics at the University of Mississippi before joining the faculty at UF. Dr. Ukeiley is an active member in many professional societies and has served on Technical Committees in both the AIAA and ASME.
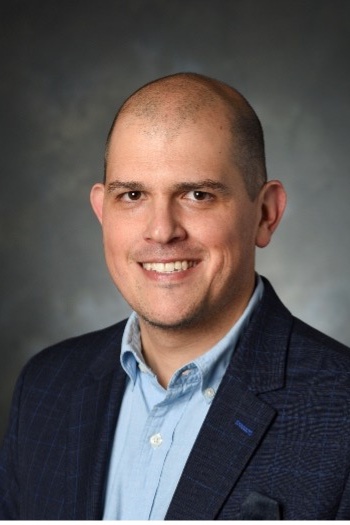
Roberto Fernández
Assistant Professor, Department of Civil and Environmental Engineering
Pennsylvania State University
Erosive processes and open research questions involving fluid dynamics
October 5, 2023
Erosion is nature's most successful artist. Its artwork is found everywhere on our planet and across many extra-terrestrial environments. It sculpts a full range of landscapes, including urban desire paths, created by human and animal footfall; meandering river canyons, created by water flows; and coastal cliffs worn away by repeated wave action. Erosion operates at many different scales, driven by fluid dynamics and its interactions with the natural and built environment. In this talk, I will discuss erosion in different contexts: (i) planform characteristics of meltwater meandering channels on ice, (ii) formation and evolution of muddy bedforms exposed to waves and currents, (iii) the disruptive nature of microplastics in riverbeds, and (iv) formation and breakage of particle bonds in clay-laden flows. As I discuss the role of erosion in the different examples, I will highlight open research questions requiring more in-depth fluid dynamics measurements that create a suite of opportunities for colleagues in the Fluid Dynamics Research Consortium at Penn State.
Biosketch
Dr. Roberto Fernández joined the faculty of Civil and Environmental Engineering at Penn State as an Assistant Professor in the Fall of 2022. He is also a faculty member of the Institute of Energy and the Environment. Before joining PSU, he was a Leverhulme Trust Research Fellow at the Energy and Environment Institute, University of Hull, UK. Dr. Fernández holds M.Sc. and Ph.D. degrees in Civil Engineering from the University of Illinois at Urbana–Champaign. His research focuses on (i) measurement technique development for water resources and geomorphology and (ii) the role of erosive processes and how they shape the landscapes around us, including sediment transport, bedrock erosion, and the formation and evolution of channels by melting (thermal erosion) and dissolution (chemical erosion). Ongoing projects include studies on the fate and transport of microplastics, morphodynamics of meltwater meandering channels on ice, and the development of Distributed Acoustic Sensing for streamflow measurements.
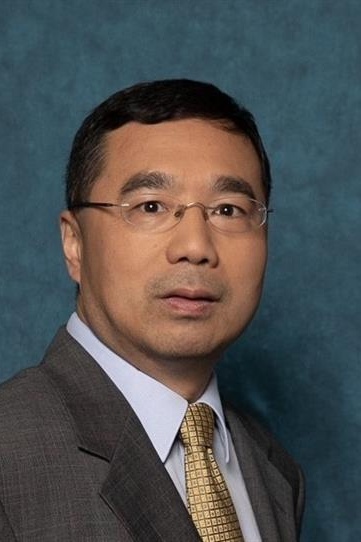
Jun Liao
Fellow Engineer, Advanced Reactor Development
Westinghouse Electric Company
Next generation Westinghouse nuclear power plants and their thermal fluids research and development
October 12, 2023
Westinghouse continues developing the next generation advanced nuclear power plants, which will provide clean and reliable electricity together with diverse applications. The technology of these plants ranges from the mature light water reactor to innovative Generation-IV liquid metal reactors. The design and key technologies of AP300 small modular reactor, eVinci microreactor and lead-cooled fast reactor will be introduced. The research and development related to thermal fluids of these reactors will be presented with a focus on the experimental facilities and analysis for the liquid lead cooled fast reactor.
Biosketch
Dr. Jun Liao earned a B.S. in Power Engineering at the Huazhong University of Science and Technology (1994), M.S. in Mechanical Engineering at Xi'an Jiaotong University (1997), and Ph.D. in Aerospace Engineering at the University of Florida (2005). He is a Fellow Engineer in the Advanced Reactor Development group at Westinghouse Electric Company. Dr. Liao is an expert in the thermal hydraulics of nuclear reactors and the development of modeling and simulation computer codes. In Westinghouse, Dr. Liao's responsibilities include developing safety analysis computer codes and methodologies for both light water and non-light water reactors. He developed and licensed the FULL SPECTRUM LOCA methodology for pressurized water reactor safety analysis. He played an important role in the development of Westinghouse's advanced nuclear power plants, including the AP1000, Westinghouse SMR/AP300, Westinghouse Lead Fast Reactor, and eVinci microreactor. Dr. Liao led the modeling and simulation development effort for the eVinci microreactor and is the safety analysis lead of the Lead-cooled Fast Reactor project. He has published over 100 journal articles and conference papers. Dr. Liao is a member of the American Nuclear Society (ANS) and American Society of Mechanical Engineers (ASME) and serves in three committees of ANS and ASME. He is an adjunct faculty in University of Pittsburgh and an associate editor of Journal of Nuclear Engineering and Radiation Science (ASME).
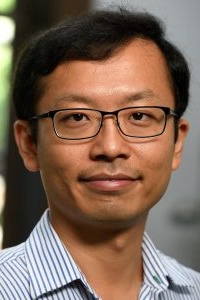
Rui Ni
Associate Professor, Department of Mechanical Engineering
Johns Hopkins University
The wrath of the small: fragmentation of bubbles in turbulence by small eddies
October 19, 2023
From milk homogenization to whitecaps in the ocean, fragmentation of bubbles and droplets in turbulence constitutes one of the most basic and practically important processes in turbulent multiphase flows. Most phenomenological models and simulations for this problem were developed based on the classical Kolmogorov–Hinze framework, even though some of the key assumptions and hypotheses in this framework have never been tested. In this talk, I will first introduce a new experimental framework that measures the geometry of deforming and breaking bubbles and their surrounding turbulence simultaneously in 3D. From this new result, I will discuss two issues that we found in the classical framework: (i) the Kolmogorov's classical theory of turbulence is not sufficient for quantifying the turbulent stresses exerted on the bubble interface and (ii) the assumption that the most relevant and energetic scale of the flow is at the bubble diameter underestimates the roles played by small eddies. Our work underlines the importance of two missing mechanisms and paves the foundation for future studies on the dynamics of polydispersed bubbles and droplets in turbulence.
Biosketch
Dr. Rui Ni is an Associate Professor in Mechanical Engineering at Johns Hopkins University and was appointed as the DOE ORISE professor in 2019. Prior to joining JHU, he was the endowed Kenneth K. Kuo Early Career Professor at Penn State University. He received his Ph.D. in the Department of Physics from the Chinese University of Hong Kong in 2011 and worked as a postdoctoral scholar at Yale and Wesleyan University. He received an NSF CAREER award in fluid dynamics, ACS-PRF New Investigator Award, Andrea Prosperetti Multiphase Flow Award and NASA Early Stage Investigation award. He is currently serving as an associate editor for the International Journal of Multiphase Flows.
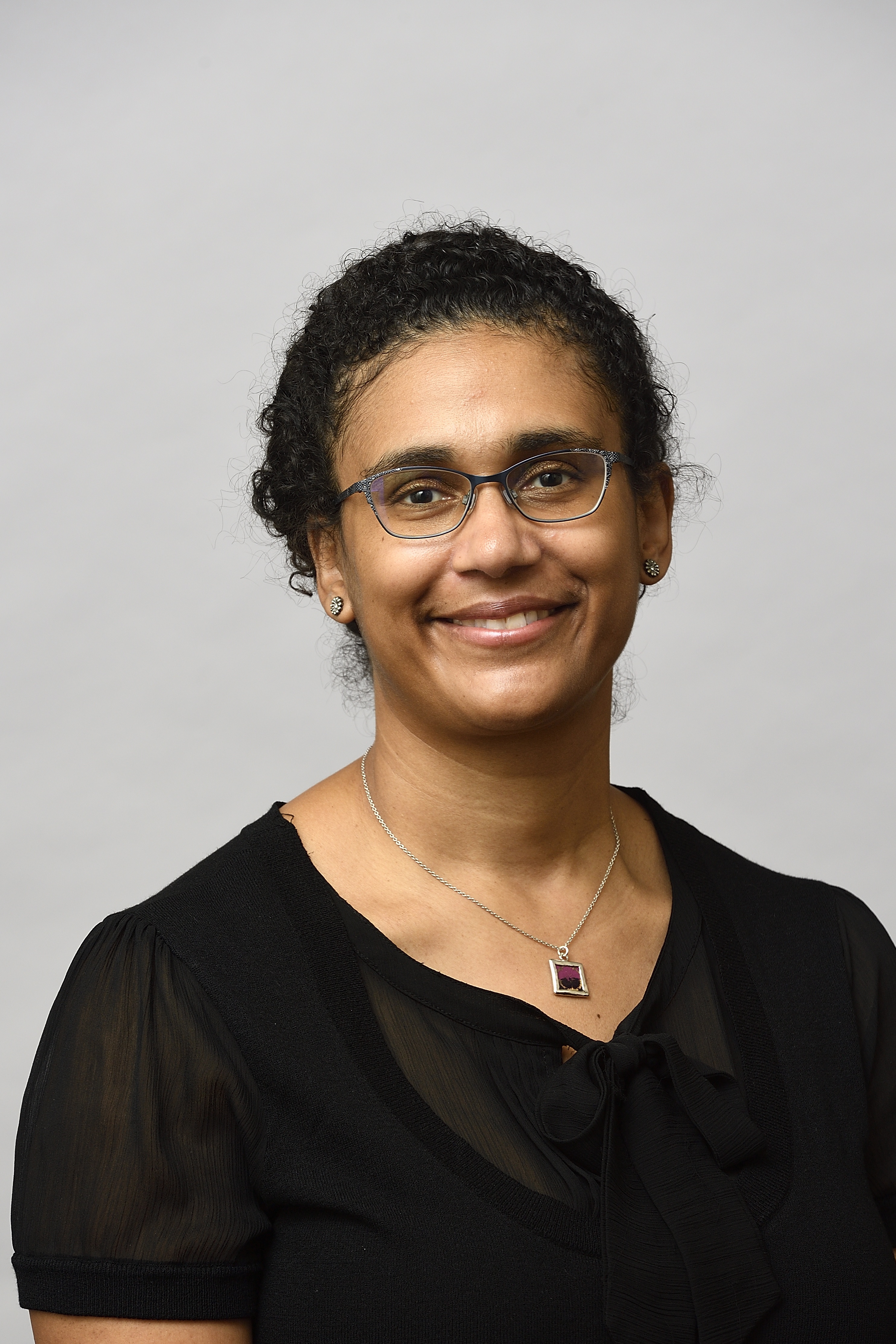
Dennice Gayme
Associate Professor, Department of Mechanical Engineering
Johns Hopkins University
Turbulence, wakes and wind farm control
October 26, 2023
The dynamics of the atmospheric boundary layer (ABL) play a fundamental role in wind farm power production, governing the velocity field that enters the farm as well as the turbulent mixing that regenerates energy for extraction at downstream rows. Understanding the dynamic interactions between turbines, wind farms, and the ABL can therefore be beneficial in improving the efficiency of wind farm design and control approaches. This talk introduces a suite of models that exploit this knowledge to improve predictions of both static and dynamic conditions in the wind farm. We then demonstrate how these ideas can be extended to a control setting.
Biosketch
Dr. Dennice F. Gayme is an Associate Professor in Mechanical Engineering and the Carol Croft Linde Faculty Scholar at Johns Hopkins University. She received her B.Eng. & Society in Mechanical Engineering from McMaster University in 1997, an M.S. in Mechanical Engineering from the University of California at Berkeley in 1998, and her Ph.D. in Control and Dynamical Systems from the California Institute of Technology in 2010. Prior to her doctoral work she was a Senior Research Scientist in the Systems and Control Technology and Vehicle Health Monitoring Groups at Honeywell Laboratories from 1999–2003. Her current research interests are in modeling, analysis and control of spatially distributed and large-scale networked systems, such as wind farms, wall-bounded shear flows, vehicle networks and power systems. She has received a number of awards for her research and teaching, including a JHU Catalyst Award in 2015, ONR Young Investigator and NSF CAREER awards in 2017, JHU Discovery Awards in 2019 & 2022, a Whiting School of Engineering Johns Hopkins Alumni Association Excellence in Teaching Award in 2020, and the Turbulence and Shear Flow Phenomena (TSFP12) Nobuhide Kasagi Award in 2022.
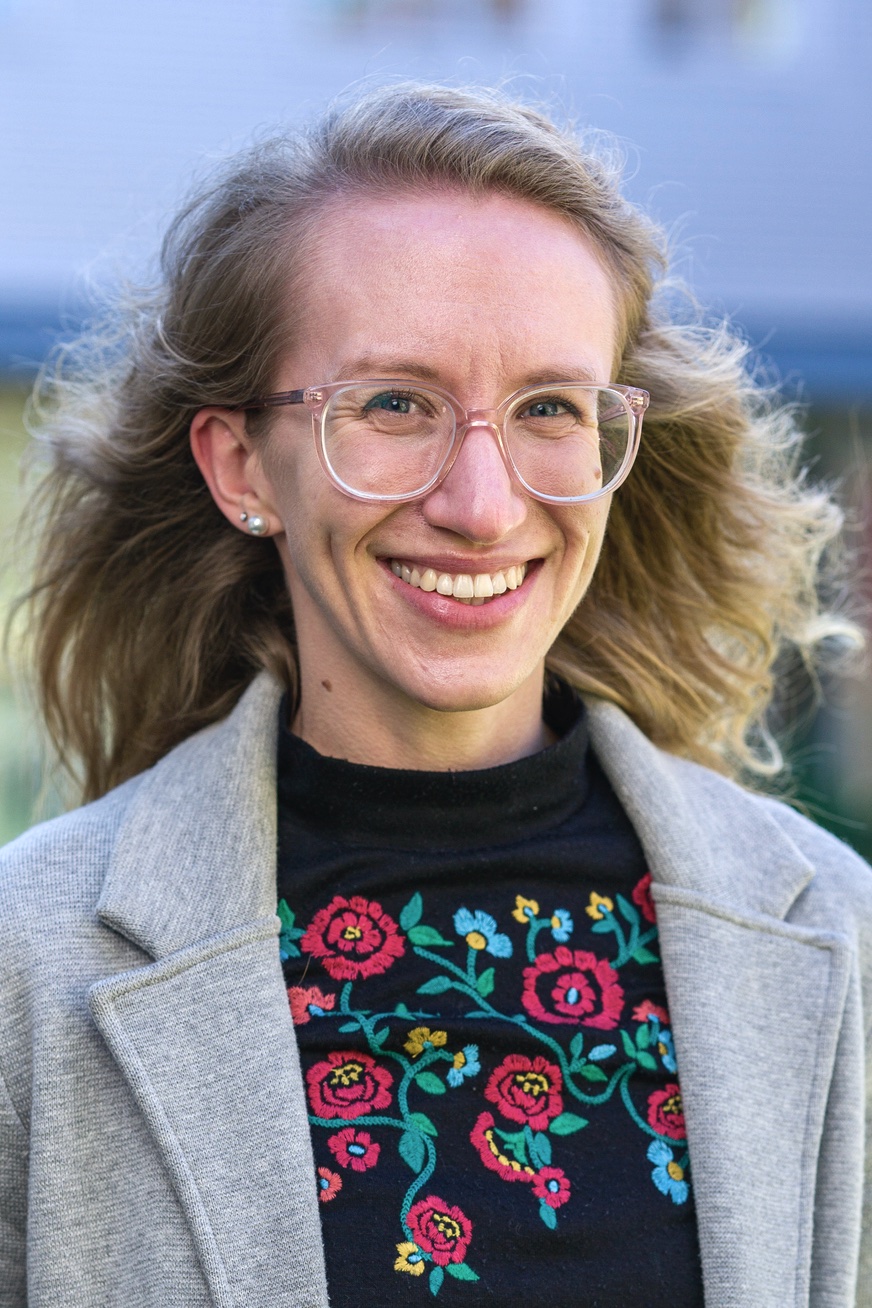
Tracy Mandel
Assistant Professor, Departments of Mechanical and Ocean Engineering
University of New Hampshire
What lies beneath: Fluid mechanics through the lens of the coastal free surface
November 2, 2023
Flow interactions at coastlines play a major role in global ocean energy and nutrient budgets. However, coastal flows can be expensive, logistically challenging, and even dangerous to study in situ. In this talk, I will cover problems that connect the water surface and subsurface dynamics in the coastal ocean, using idealized laboratory experiments to understand the physics of these systems. First, I will discuss the first step in remotely characterizing seagrass meadows by studying the overlying water surface. Flow through a seagrass bed can generate large overturning vortex structures, which cause small perturbations in the free surface slope. Using laboratory experiments, we develop a parameterized model to reconstruct within-canopy velocity profiles solely from water surface measurements, suggesting that in some environmental flows, the subsurface hydrodynamics and geometry may be predicted by measuring the water surface behavior alone. Second, I will examine the dynamics of turbulent buoyant plumes, such as those that might emerge at the base of a marine-terminating glacier, including preliminary work that quantifies the surface expression of these buoyant plumes.
Biosketch
Dr. Tracy Mandel is an Assistant Professor in Mechanical Engineering and Ocean Engineering at the University of New Hampshire. Her research explores the natural physical processes that occur at ocean margins in order to better protect, restore, and consider these systems in engineering decisions. Tracy received her B.S. from Cornell University and her M.S. and Ph.D. from Stanford University, and she was awarded the 2018 Lorenz G. Straub Award for most meritorious doctoral dissertation in hydraulic engineering, ecohydraulics, and related fields.
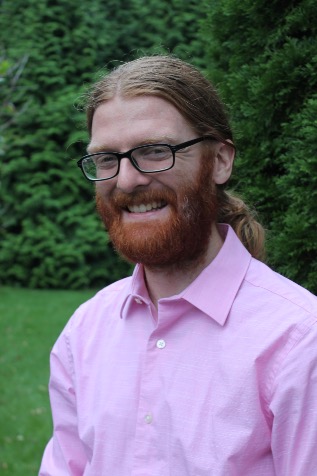
Ryan Murray
Assistant Professor, Department of Mathematics
North Carolina State University
Vorticity, symmetries, ill-posedness and singularity formation in incompressible fluids
November 9, 2023
Many fundamental fluid flows can be described succinctly in terms of fluid vorticity. However, often the vorticities associated with physical flows are not smooth: examples include shear layers, spirals, and vortices. Many mathematical challenges come as a consequence of non-smooth vorticities. In this talk, I'll give an overview of some of these mathematical questions. In particular, I'll describe recent mathematical work which utilizes symmetry solutions to better understand two-dimensional incompressible flows: these works describe fundamental ill-posedness of flows with singular vorticities, as well as a universal tendency for Lagrangian representations to become less smooth over time. This talk will aim to be accessible to a broad audience interested in fluid dynamics. This represents joint work with Alberto Bressan, Galen Wilcox, Ayman Rimah, and Tarek Elgindi.
Biosketch
Dr. Ryan Murray is an Assistant Professor in the Department of Mathematics at North Carolina State University. Previously, he was a Chowla Assistant Research Professor in the Department of Mathematics at Penn State University (2016–2019), and he earned his Ph.D. at Carnegie Mellon (2016). Ryan's work focuses on using tools from mathematical analysis to understand a wide range of applied problems, including problems from fluid dynamics and data science.
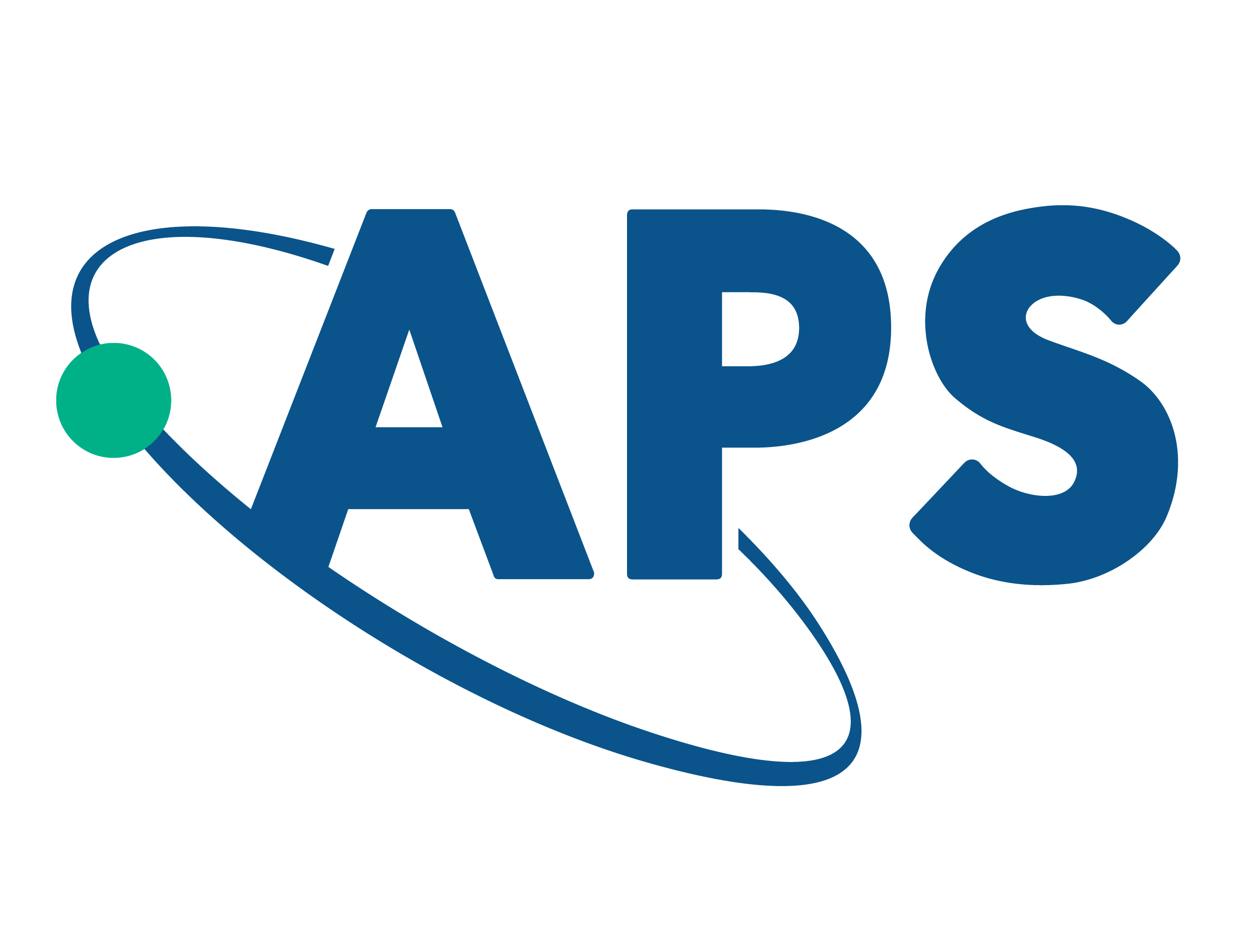
APS Prep Session
November 16, 2023
Application of machine learning in non-Newtonian flows
Dibyajyoti Chakraborty, College of Information Sciences and Technology
With the abundance of available data and advancements in machine learning, there is a growing need to shift toward data-driven and optimization-based approaches for solving problems that were traditionally solved using CFD techniques. Neural networks, especially, offer great potential in capturing the chaotic nature of dynamic phenomena. On the other hand, established physical laws serve as benchmarks of knowledge, validating the results obtained through computational and data-driven methods. In this work, we have implemented the latest Physics Informed Neural Networks (PINN) to solve non-Newtonian flows and compared their accuracies with theoretical/numerical solutions and available experimental data. Additionally, we have used numerical simulations to generate data for non-Newtonian flow over spheres under a range of operational parameters. We have shown how data from numerical experiments can be used to predict properties, like drag, which would otherwise have much higher computational costs. Finally, we have demonstrated that machine learning could be used to optimize the shape of objects in non-Newtonian flows without the requirement for performing numerical simulations over the entire domain of different shape parameters.
Reversibility, path-dependence, and memory of a creeping triple-phase contact line
Ashbell Abraham, Department of Physics
The contact line around a water drop on a horizontal surface has an irregular shape that does not relax to equilibrium, revealing the disorder of the solid substrate beneath. We show that the contact line has a detailed memory of its history of motion on the surface. To form a memory, we start with an initial volume of water and "train" the contact line with slow imbibition and drainage cycles at constant volume amplitude until its motion becomes periodic. Reducing the volume amplitude drastically changes the shape of the contact line when it returns to its starting volume, but when driven again with only one cycle at the training amplitude, it transitions back to its steady state. Driving above the training amplitude erases the memory making the steady state inaccessible. This behavior is reminiscent of return-point memory, a phenomenon best known in ferromagnets. Return-point memory, and the evolution to steady state, can give insight on how contact line hysteresis and the memory of its motion arise, offer a framework to study its reversible-irreversible transitions, and provide a comparison of this system to others that are far from equilibrium.
The hydrodynamic properties of unconventional surface roughness
Shyam Nair, Department of Mechanical Engineering
Surface roughness is characterized by its statistics such as the average height, ka, the root-mean-square, krms, the skewness, Sk, etc. A survey of the roughness in the existing literature shows limited work on roughness with low Sk and high krms/ka, which is the focus of this talk. By controlling the spacing, height, and arrangement of rectangular protrusions and pits, such rough walls are constructed. Direct numerical simulations (DNSs) are conducted to study their hydrodynamic properties. The data suggests that Sk has a minor effect on the equivalent sandgrain roughness height (ks) as pits, that contribute to negative Sk, do not significantly contribute to drag. Furthermore, the roughness arrangement, which is not captured by single-point roughness statistics, has a significant effect on ks. Attempts in developing correlation-type rough-wall models show that the predictive power of a rough-wall model depends on how well the input space distinguishes the rough walls in the calibration dataset. Moreover, the importance of roughness statistics depends on the rough walls under consideration. These findings suggest that a universal rough-wall model might not be possible, and models for specific types of roughness might be more practical.
Reconstructing complex flows from inertial Lagrangian particle tracks
Ke Zhou, Department of Mechanical Engineering
Particle tracking velocimetry (PTV) is widely used to reconstruct 4D flow states from Lagrangian particle trajectories, a.k.a. "tracks", assuming that particles faithfully follow the flow. However, particles can lag the flow or travel ballistically due to rapid acceleration, large temperature gradients, strong body forces, etc., complicating the interpretation of PTV data. We report a novel method to simultaneously reconstruct unsteady flow states and individual particle properties (e.g., size, density, effective response time, ...) from inertial tracks. To do this, we use a neural-implicit particle transport model to predict PTV tracks as a function of estimated flow states and particle properties. The flow states and tracks are parameterized by physics-informed neural networks (or similar). Optimizing an objective loss comprising a PTV data loss, Navier–Stokes residuals, and particle transport residuals yields tracks that match the data, physically-plausible flow states, and estimates of the unknown particle properties. We demonstrate this approach using synthetic tracks of inertial particles carried by laminar and turbulent flows. To the best of our knowledge, we report the first unsteady flow reconstructions from inertial tracks as well as implicit PTV-based particle sizing.
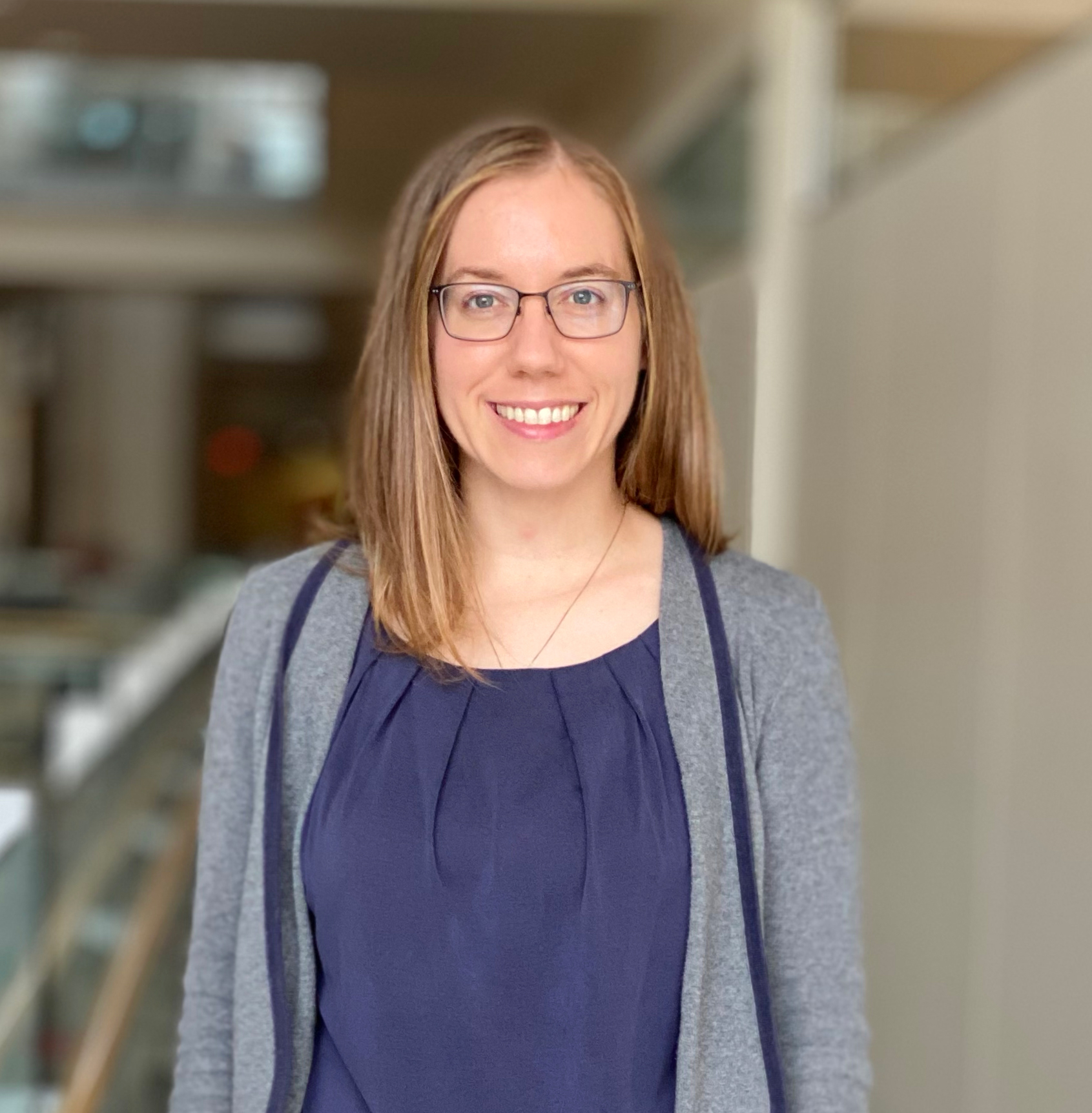
Colleen Witzenburg
Jane R. and John G. Mandula Assistant Professor, Department of Biomedical Engineering
University of Wisconsin–Madison
Using reduced order computational fluid mechanics models to predict and quantify cardiovascular growth and remodeling during development
November 30, 2023
Congenital heart defects, failures of the heart to develop properly in utero, are the most common birth defect, occurring in approximately 1% of live births. Clinical management of infants and children with these defects often involves estimating how hemodynamics and heart and vessel dimensions will change with growth and development. Previous computer models have successfully predicted cardiac growth and remodeling in adults with various pathologies. However, applying these models to infants and children is complicated by the fact that they also undergo normal, somatic cardiac growth and remodeling.
In this talk, I will present two reduced order computational fluid mechanics models to predict and quantify adverse cardiovascular growth and remodeling. The first model successfully simulates clinical measurements of pressures, ventricular and atrial volumes, and ventricular thicknesses within two standard deviations of multiple studies for healthy infants from birth through three years of age. Then, using this model we simulated coarctation of the aorta, a common congenital defect that typically presents as a localized narrowing of the aorta. In these simulations we predicted the temporal course of increases in systemic blood pressure, left ventricular thickness, and left ventricular volume following trends in clinical data. The second model was used to simulate pressure, flow, and cross-sectional area throughout the pulmonary arteries of individual animals with left branch pulmonary artery stenosis, a narrowing of the artery that supplies blood to the left lung. The reduced order model was composed of the large pulmonary arteries, whose length and radius were specified from animal-specific imaging, and the distal vasculature, which was simulated by a three-element Windkessel model at the outlet of each terminal vessel. Remodeling in the large pulmonary arteries and distal vasculature estimated via model fitted parameters was consistent with terminal histology. Ultimately, our computer models enable a greater understanding of somatic and pathologic cardiovascular growth and remodeling due to stenotic congenital heart defects. The flexibility and computational efficiency of these models allow for rapid analysis of pathological mechanisms affecting cardiac growth and hemodynamics.
Biosketch
Dr. Colleen Witzenburg is the Jane R. and John G. Mandula Assistant Professor in the Biomedical Engineering Department at the University of Wisconsin–Madison. She also has affiliate appointments in the Mechanical Engineering Department and the Department of Pediatrics, and she is a member of the Cardiovascular Research Center and Carbone Cancer Center. Dr. Witzenburg earned a B.S. in Mechanical Engineering from Iowa State University and a Ph.D. in Mechanical Engineering from the University of Minnesota. She then completed postdoctoral research at the University of Virginia. Her lab group, the Cardiovascular Biomechanics Lab, has been awarded grants from the National Science Foundation, the American Heart Association, the Children's Heart Foundation, the Wisconsin Alumni Research Foundation, and the UW Institute for Clinical and Translational Research.

Michelle Driscoll
Assistant Professor, Department of Physics and Astronomy
Northwestern University
Microrollers meet boundaries: trapping, structure, and more
December 7, 2023
Driven suspensions, where energy is input at a particle scale, are both models for understanding general principles of out-of-equilibrium self-organization, and also materials with enormous near-term applications potential. My work is focused on magnetically-actuated suspensions; I study how these materials assemble into dynamical structures, as well as how they interact with obstacles and boundaries. While the model system we employ is very simple (spinning particles in water), the strong hydrodynamic interactions between individual particles and with nearby boundaries lead to a rich array of emergent structures. Recently, we found that even simple modulations to nearby boundaries can have dramatic consequences: a single post-shaped obstacle can act as a hydrodynamic trap, and capture a passing particle. Moreover, the strength of this trapping can be easily be tuned by adjusting either the obstacle curvature or the particle-obstacle repulsive potential. This work demonstrates the complexity of this dynamical system: microrollers can become trapped by an obstacle, this trapping is stochastic, and most surprising, it is enabled by and not destroyed by thermal fluctuations. I will close by discussing how we are using this work to understand suspension transport in more complex structured environments, as well as how we can use these particles to reconfigure their local environment.
Biosketch
Dr. Michelle Driscoll is a soft condensed matter experimentalist, and her research lies at the junction between soft-matter physics and fluid dynamics. The Driscoll lab focuses on understanding how structure and patterns emerge in a driven system, and how to use this structure formation as a new way to probe nonequillibrium systems. The lab studies emergent structures in a diverse array of driven systems, from the microscopic to larger-scale. By developing a deeper understanding of patterns and structures which emerge dynamically in a driven material, we can learn not only how these structures can be controlled, but also how to use them to connect macroscopic behavior to microscopic properties. Before coming to Northwestern, Prof. Driscoll was a postdoctoral associate at New York University, working with Paul Chaikin in the Center for Soft Matter Research. She completed her Ph.D. in 2014 with Sid Nagel at the University of Chicago.